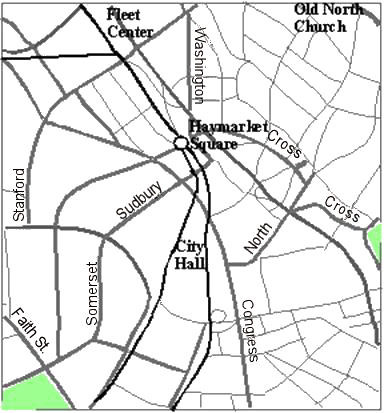 |
Figure 1. A schematic map showing the road complexity of downtown
Boston.
|
Driving successfully through the streets of Boston can be an
enormous challenge. This challenge is less a function of the other
drivers, who at times may be aggressive and creative in road rule
interpretation, but rather is mainly due to the complexity of the
environment. Traffic rotaries, one-way streets, and road
configurations that rarely align with grid-like patterns can create
navigation nightmares (see Figure 1). This notion is not lost on
the local populace: Urban legend purports a city rotary designed
only with one-way streets feeding into the rotary. Even recent
attempts to alleviate traffic difficulties have created further
challenges. Consider the infamous Big Dig, a major ongoing
construction project designed to revamp both above- and below-ground
traffic routes through the city, alleviating traffic snarls through
the heart of Boston. Work on the Big Dig frequently creates
extended detours through the already existing maze of streets and
thoroughfares. These detours add to the challenge of navigating
these complex neighborhood thoroughfares since they force drivers to
update their mental representation of the city to accommodate
uncertain changes. Since a detour can be experienced at virtually
any point along one's normal route, accessing and updating one's
mental representation must be done out of some previously learned
sequence. Such experiences are not limited to those involving
Boston (although some Bostonians might take issue with that notion);
consider the last time you negotiated your way around a construction
detour or traffic jam, guessed where to go next given an
underspecified set of directions, or tried to find your way in your
favorite city after being away for a long period of time (and
finding much has changed). How do we update our memories of space
to successfully navigate through varying, complex, and sometimes
changing environments?
Some of the earliest, preliminary work on updating spatial
representations comes from Tolman's (1948) research on how animals
mentally represent environments (Tolman, 1948; Tolman & Honzik, 1930). In
these studies, rats learned to navigate a maze and, with sufficient
training, could generate novel shortcuts through the environment.
Tolman described these mental representations or memories as
cognitive maps. While the nature and definition of such
mental representations remains a matter of debate [for definitional
and interpretive discussion see Bennett (1996)], rats' use of novel
shortcuts suggests that the environment was represented in a form
that could be accessed "out of sequence"; that is, in ways beyond
that which had been learned. In other words, animals can use
their mental representations of environments to do more than follow
a well-worn path. Some believe that human navigators
demonstrate even greater flexibility than animals like rats in their
ability to access information "out of sequence" from their cognitive
maps (e.g., Taylor, Naylor, & Chechile, 1999; Taylor & Tversky, 1992b). This
flexibility includes the mental manipulation of spatial information
based on different orientations, perspectives, and geographic
scales, from a wide variety of acquisition sources (maps,
navigation, and spoken or written language). (See
Schmajuk & Voicu, this
volume.)
While
early work on spatial representations relied on non-human
experimentation, current examinations of spatial cognition involve
investigations of both human and non-human species. Can we derive
general principles from such work? Indeed some human spatial
processing is achieved through neural circuitry shared with
non-human animal species, particularly the hippocampus (e.g.,
McNamara & Shelton, 2003; O'Keefe & Nadel, 1978; Wang & Spelke,
2002; also see Mizumori & Smith,
this volume). Beyond these structural similarities there are, perhaps not
surprisingly, some functional similarities between humans and
non-humans' use of a cognitive map (e.g., O'Keefe & Nadel, 1978).
For example, both humans and non-humans sometimes use an
environment's shape to guide navigation (Gallistel, 1990; Gouteux &
Spelke, 2001; Hermer & Spelke, 1994). Even more compelling, and for
some individuals quite controversial, is the idea that there may be
similar underlying processes or activities involved in constructing
and accessing human and non-human spatial representations. (For a
discussion of this issue see Wang & Spelke, 2002.)
There are also important differences between human and
non-human animals' spatial representations. Perhaps most
importantly, humans, unlike non-humans, have evolved mechanisms to
represent spatial information symbolically (Gattis, 2001; Gauvain,
2001; Glasgow, Narayanan, & Chandrasekaran, 1995; Plumert, Ewert, &
Spear, 1995;
Taylor & Tversky, 1992a; Uttal, 2000). These representational
mechanisms allow humans to acquire spatial information from means
other than through direct experience. Indeed, humans gather a
substantial amount of spatial information from symbolically mediated
sources, such as maps, diagrams, and verbal descriptions.
Importantly, cognitive maps constructed from such indirect, symbolic
sources have been shown to differ from those derived from direct,
navigational experience in some ways (e.g., Thorndyke & Hayes-Roth,
1982) and yet similar in other ways (e.g., Taylor et al.,
1999).
The
evolutionary mechanisms in humans that allow for symbolic spatial
representations may also allow for ready flexibility in thinking
about and using spatial information beyond the activities exhibited
by non-human animals. By flexibility, we mean the ability to use
spatial information in novel ways that transcend direct experience,
including updating and manipulation. The short-cuts shown by
Tolman's (1948) rats certainly transcend direct experience to a
degree. However, this flexibility is extended in humans with
symbolic representations, as the activities involved in building
such representations bear little surface resemblance to the direct
experience of navigating through an environment. A map uses visual
and verbal symbols, often to depict an environment that is more vast
and complex than what can be experienced from a single vantage
point. Maps give direct information about spatial relationships
between locations, but rarely provide spatial information relative
to the individual and generally take a perspective above rather than
within the environment (MacEachren, 1994, 1995). Verbal or
text-based spatial descriptions can rely solely on language; thus
many spatial relations are not explicitly provided in the linguistic
stimuli and must be inferred. These descriptions may take either a
within-environment (route) or above-environment (survey) perspective
and generally omit metric detail to increase comprehensibility
(Taylor & Tversky, 1992b). Yet, humans demonstrate a facile ability
to construct cognitive maps from both cartographic maps and spatial
descriptions (Taylor & Tversky, 1992a, 1992b, 1996; Thorndyke &
Hayes-Roth, 1982).
Even the concept of a cognitive map implies flexibility in thinking
about spatial information since a map, whether it be physical
or mental, takes a different perspective on an environment than does
actual navigation (the most common form of direct experience). Of
course, there are numerous ways in which humans interact with
spatial environments and numerous ways in which environments relate
to one another and can be integrated. These factors can combine to
create a variety of situations for humans to experience spatial
information, indeed more than one could hope to comprehensively
count.
In
this chapter, we will examine evidence on updating and manipulating
spatial representations across some of these situations, drawing
conclusions about general cognitive mechanisms that are involved
with some types of spatial mental representation use. In
particular, we will concentrate our discussion on the ways in which
we build and update spatial representations based on (1) direct
experiences (e.g., navigating an environment) and (2) more symbolic
experiences (e.g., studying a map of an environment or reading a
spatial description), with a particular focus on situations that
require using spatial information "out of sequence." Our ability to
comprehend this latter, symbolic form of experience in a wide
variety of contexts may be what distinguishes us from non-human
animals. Within the human animal, though, many of the processes and
products of spatial cognition may be invariant across symbolic and
direct experiences in human information processing.
As
we have just described, one method for acquiring spatial
representations involves direct experience navigating an
environment. Representations based on navigation have been
suggested to be orientation-free, meaning that information can be
accessed from the representation equally well from any orientation
(e.g., Sholl, 1987). In any navigation experience, one experiences
the environment from many different orientations and vantage
points. Every turn results in a new orientation; every step results
in a new vantage point. The idea of an orientation-free mental
representation comes from the need to integrate experiences based on
multiple views acquired during navigation. Only through integration
can one more fully represent the whole environment, particularly
environments that cannot be experienced from a single orientation
(e.g., a building, a college campus, or a town). Thus, an important
issue is how a spatial representation is constructed or built from
these individual "snapshot" views of space. While the idea of
orientation-free representations from navigation may make intuitive
sense, empirical evidence has not always concurred with those
intuitions.
For
example, what happens when an environment is experienced from more
than one, but still a limited number, of orientations? Shelton and
McNamara (1997) had participants learn an object array from two
views, with the goal of determining whether multiple views would
facilitate the construction of an orientation-free representation.
Contrary to the orientation-free idea, their results suggested that
people maintained two orientation-dependent representations - one
for each studied view. In other words, their participants'
representations were more like mental snapshots. Furthermore, Diwadkar
and McNamara (1997) showed that when tested on novel views,
participants needed to reconcile the novel view with the studied
view, which led to cognitive difficulty as indicated by response
time differences.
Yet
while performance appears orientation-dependent with multiple,
limited views, the underlying mental representation does not seem
necessarily tied to actual viewing orientations. Mou and McNamara
(2002) had participants attend to an intrinsic axis within an object
layout. The intrinsic axis is one internal to the array and in this
case was created through the alignment of objects within the array.
The intrinsic axis was either congruent with or displaced from the
participants' actual viewing direction. Results showed an
orientation-dependent representation tied to the intrinsic
rather than the viewing axis. Pointing accuracy was actually
greater when activities were aligned with the intrinsic axis,
regardless of facing direction. Additionally, novel headings
orthogonal to this axis led to more accurate pointing judgments than
other headings. So, this study shows orientation-dependence rather
than orientation-independence as a function of direct spatial
experience
(A)
(B)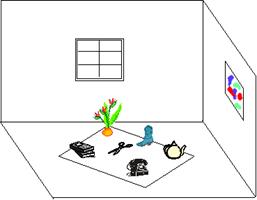 |
Figure 2. A spatial array based on
Shelton and McNamara (2001a) wherein (A) local reference frame is
aligned with the global reference frame defined by the room and
wherein (B) local reference frame is misaligned with the global
reference frame defined by the room.
|
In further examinations of orientation-free representations, Shelton
and McNamara (2001a) had participants learn a layout of objects from
one, two, or three viewpoints. The viewpoints were either aligned
(see Figure 2A) or not aligned with a salient, environment-defined
reference frame (see Figure 2B), in this case the walls of the
room. Results indicated that if a viewpoint aligned with the
environment reference frame, other actual viewpoints were not as
strongly represented. Imagine that David learned the object array
in Figure 2A standing on the side of the mat between the phone and
flower vase. Holly, in contrast, learned the object array in Figure
2B, standing on the side of the mat between the flower vase and the
stack of books. The actual viewpoint experienced by Holly would not
be represented as strongly as the one experienced by David because
Holly's was not aligned with the walls of the room.
In
a cross-modal version of this task, Shelton and McNamara (2001b) had
participants view a table-top object layout and then manually,
without visual access, reconstruct it from either the same or a
rotated orientation. They found better visual memory for the
manually reconstructed viewpoint than for the visually experienced
one. Taken together, these findings indicate that for object layouts
experienced from several, but still a limited number of viewpoints,
participants represent the layout in orientation-dependent ways.
The fact that the orientation dependency is not necessarily tied to
the viewing orientation suggests that humans possess the cognitive
facility and flexibility to develop and update mental
representations that differ from perceptual experience. Our
cognitive apparatus appears to take advantage of a variety of different
reference frames (e.g., egocentric, intrinsic, or environmental),
depending on availability in the environment, any of which could
form the basis of orientation-dependency in a mental
representation. It is important to note that in the studies just
reviewed, the entire spatial array or environment could be viewed
from a single vantage point.
Unlike a spatial array that can be viewed completely from a single
vantage-point, many real-world environments, such as a college
campus, cannot be completely experienced from a single viewpoint.
How does the variety of viewpoint experiences we have with these
environments impact the resultant mental representation? McNamara,
Rump, and Werner (2003) investigated mental representations
developed from navigation through a large-scale, real-world
environment (the Parthenon and its surrounding park in Nashville,
Tennessee). Participants navigated through the environment on a
path that was either aligned or misaligned with the walls of the
Parthenon. Participants in the aligned path condition used the
structure of the Parthenon to guide their representations, showing
increased pointing accuracy for heading directions aligned with the
structure's walls. Those in the misaligned condition relied on an
obvious landmark (a nearby lake) as a central reference point in
their mental representation. This finding suggests that mental
representations involve the use of reference frames as defined by
the environment, but that are also influenced by our direct
egocentric experience with that environment.
Navigation within an environment actually involves two types of
changes - rotation and translation. A simple rotation would involve
turning in place; a simple translation would involve walking
forward. These two types of changes are obviously used in concert
during navigation. Rotational or orientation changes seem more
difficult to update than translation changes (Easton & Sholl,
1995; Presson & Montello, 1994; Rieser, 1989). The ability to
update also appears tied to actual locomotion. Self-movement
facilitates automatic updating of spatial relations (Farrell &
Robertson, 1998). Rieser, Garing, and Young (1994) found that young
children could imagine perspective changes when they were tied to
physical actions, but not when they were only imagined. In
contrast, adults could imagine perspective changes, but were slower
to do so than when changes involved actual physical motion.
Furthermore, errors in updating an imagined heading appear tied to
perceptual representations of the body (Avraamides, Klatzky, Loomis,
& Golledge, 2004) and error is reduced when body-based information is available
(Waller, Loomis, & Haun, 2004).
Orientation-free representations based on navigation would seem to
afford the most flexibility for updating "out of sequence." The
intuition underlying the idea of orientation-free representations
comes from the integration of many and varied experiences with an
environment in terms of both orientation and vantage point, with
changes in position taking place through both rotation and
translation. Yet, as reviewed above, the empirical evidence has not
provided consistent support for orientation-free representations.
Sholl and Nolin (1997), based on five studies, suggest that
orientation-free performance is only evidenced under a certain set
of conditions and if any one of those conditions is not met,
performance will be orientation-dependent. The conditions outlined
include a horizontal viewing angle during encoding, a room-sized test
space, and "on-path" testing. Some of these conditions suggest that
spatial perspective plays a role in spatial representation use,
particularly when considering how such representations are used "out
of sequence."
When considering how people gather and use spatial information,
particularly symbolic information sources, a shift in perspective is
frequently involved. Navigation necessarily involves a
within-environment or route perspective. This would map on to the
horizontal viewing angle suggested by Sholl and Nolin (1997). As
one moves in the environment, landmarks are viewed relative to one's
current position in the environment. Spatial experiences other than
direct navigation can differ with respect to perspective. For
instance, spatial descriptions can also relate a route perspective
while maps, in contrast, present a spatial perspective from above,
also known as a survey perspective. Spatial descriptions can also
adopt a survey perspective, or can even mix survey and route
perspectives (Taylor & Tversky, 1996).
 
When using symbolic sources, shifts between route and survey
perspectives are not uncommon. For example, someone may draw a map
of an area they have only experienced through direct navigation,
such as one provided in invitations to dinner party guests. The
guests, in turn, must shift between spatial perspectives in the
opposite direction by using the map to navigate to the party. That
is, they use a survey perspective provided by the host to help
configure a route perspective that will lead them from their home to
the party. Although these perspective shifting tasks are
commonplace, they are anything but cognitively simple.
To
understand the cognitive activities involved in switching or
updating across perspectives, one must understand the mental
representations involved. Is a mental representation a function of
the perspective learned? Based on the existing research, the answer
is unclear. Some findings support different representations from
maps and navigation (e.g., Thorndyke & Hayes-Roth, 1982). For
example, Sholl (2000) argues that the coordinate systems used to
access spatial information are separable and are derived from the
learned perspective. Navigation uses a body-centered system and
maps use an object-centered system. Thus, people would have
different representations structured by the perspective learned. As
discussed above, the differences may relate to having an
orientation-free mental representation following navigation and an
orientation-specific one following map study (Sholl, 1987).
Opposing views suggest either no perspective-based differences in
mental representations (McNamara, Hardy, & Hirtle, 1989) or changes in
mental representations only resulting from extensive experience with
an environment (Golledge & Spector, 1978; Thorndyke & Hayes-Roth,
1982). This latter explanation has been embodied in computational
models of spatial memory wherein iterative manipulation of route
information leads to accurate survey perspective inferences and
better updating "out of sequence" (Kuipers, 1978; Leiser &
Zilbershatz, 1989).
Why
do some studies find perspective-based differences and others do
not? Several factors may account for these seemingly contradictory
results, all of which have implications for how individuals use
spatial models across perspectives. The representational strategy
adopted may rely on situational factors, for example whether
landmarks can be reliably recruited. Foo, Warren, Duchon, and Tarr
(2005) found that participants relied on landmarks when available,
but if landmarks appeared unreliable, they would switch to a rough,
survey-based representation. In fact, they appeared unable to find
novel paths through environments that were devoid of landmarks.
Beyond map characteristics, individuals' goals for a spatial task
can also influence the mental representation people construct and
apply. Taylor, Naylor, and Chechile (1999) obtained
representational differences depending on both how people learned an
environment and what they expected to do with their knowledge. A
survey-perspective goal led to better performance on
survey-perspective tasks while a route-perspective goal led to
better performance on route-perspective tasks. Additionally, the
amount of experience an individual accumulates in an environment may
influence spatial representations. People seem to develop mental
representations with more survey perspective characteristics with
longer exposure to the environment. Combining these various
characteristics, Thorndyke and Hayes-Roth (1982) found perspective
differences based on learning format, either map or navigation.
Navigation learning led to an improved ability to orient to unseen
locations and estimate route distances; map learning lead to
superior judgments of relative location and straight-line
distances. However, the map learning advantage disappeared for
people with extensive navigation experience.
Taken together, these results indicate that cognitive flexibility for
thinking about the same spatial information from different
perspectives increases with increased experience, whether this
experience is based on actual navigation or mentally derived from
specific learning goals. The results also indicate that people can
use, to their advantage, the information available in a variety of
ways and "out of sequence." Landmarks may be ideal for navigating,
but when absent, people appear to recruit and use the information
that is available, even when this requires switching perspectives.
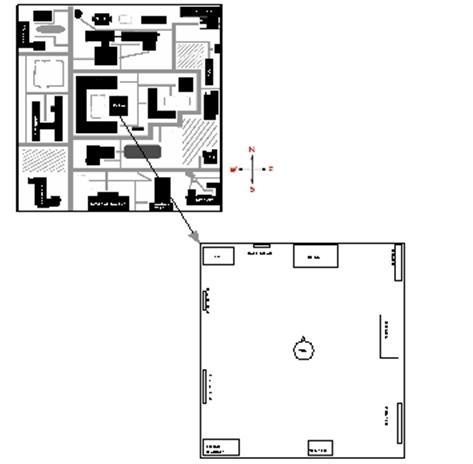 |
Figure 3. A map depicting environment
nesting. Insert portion of map illustrates a room within building
on this college campus (Bilge & Taylor, 2006).
|
Real-world environments have nested units
(see Schmajuk & Voicu, this volume). Rooms are positioned
within buildings, buildings are located within towns, towns appear
within counties, etc. (see Figure 3). While evidence suggests that
people continuously update their spatial representations as they
move (Wang & Spelke, 2000) through a process referred to as spatial
updating (Wang & Brockmole, 2003a, 2003b), whether such updating
simultaneously incorporates these various levels of nesting is not
clear. Extant evidence suggests that it does not. Environmental
objects appear to be egocentrically updated one by one as opposed to
calculating one's position within the environment as a whole (Wang &
Spelke, 2000). Thus, the representational system selects the most
important objects or locations.
Which objects may be selected as
"most important"? From the point
of view of navigation, objects in the immediate surround, which may
be bumped into or tripped on, would seem important to track.
Consistent with this, Wang and Brockmole (2003a) found automatic
updating of the immediate environment, but effortful updating of the
more remote environment. In other words, automaticity of updating
was asymmetric. When asked to update the remote environment, people
automatically update the immediate environment; when asked to update
the immediate environment, they do not automatically update the
remote environment. The same asymmetry has been shown between real
and imagined environments wherein the real environment was
automatically updated and the imagined was not (Wang, 2004),
supporting the idea that updating is based on importance. This
asymmetry in updating, favoring proximal environments over
larger-scale, more distal ones, illustrates cognitive efficiency and
selectivity. The finding, though, does not mean that people
cannot update across scales. In fact, through their procedure,
Wang and Brockmole (2003a) showed directly that when asked, people
can, with effort, update the distal, larger scale environment, even
when physically situated within the proximal environment. Furthermore,
Bilge and Taylor's (2006) work suggests that updating across scales
is more likely to occur after learning from a map, as opposed to
direct experience.
One
uniquely human symbolic system that can convey spatial information
is language. By language, here, we are not referring to the diverse
methods that non-human animals have for conveying information (e.g.,
scent, posture, movement, or vocal calls). What we mean by language
is the use of verbal information, whether spoken, written, or
signed, to convey idea units or concepts. This human-specific form
of language is a direct and effective means of detailing spatial
information to others. For example, we have all experienced
situations in which a passing driver rolled down his window, asking
for directions. Using spoken language (e.g., "Turn left at the next
stoplight."), sign language, and perhaps even written tools (e.g.,
jotting down the name of a street), the driver can be directed to a
destination.
Language
can also be used to describe environments that an individual may
never actually visit at any point. Indeed some of these
environments may not actually exist, except in fiction.
Descriptions of locations in fictional novels often detail
relationships between objects, landmarks, and other geographic
locations to such a degree that the reader can develop a fairly
complex representation of the description. The reader will never
have the opportunity to act on that representation and negotiate
those environments (e.g., the terrain described in The Lord of the
Rings, the locations described in a Tale of Two Cities, or the hotel
depicted in Psycho). Nevertheless, the processes involved in
constructing and updating such a representation bear many
similarities to those necessary for building a spatial
representation while navigating or studying a map. One difference,
of course, is the degree to which an individual has physical,
kinesthetic experience in those locations (similar issues arise with
virtual environments). Such a difference, though, does not suggest
that the underlying cognitive processes necessary for building,
updating, and applying such a representation would be different in
any systematic way.
Language,
then, is an incredibly effective tool for informing the construction
and updating of spatial representations. Language, in a way that is
not intended as specific only to spatial experiences, has been
described as a set of instructions that instruct the comprehender as
to what, and how, information should be represented in memory (e.g.,
Givon, 1992). For example, references such as "he" and "they"
indicate that prior information from the discourse should be
reactivated and kept in current focus to ensure understanding of the
current linguistic input. Thus, linguistic forms such as simple
grammatical constructions (e.g., pronouns) all the way up to direct
statements (e.g., "This next bit is important, so pay attention.")
provide information about what readers should track and update. In
situations involving spatial descriptions, processing instructions
can include, as examples, spatial prepositions such as "next to,"
"toward," "go through," and so on. These prepositions indicate the
direction (and type) of movement, the relationships between
important landmarks and features of the environment, and the
intended goal of spatial activity. Spatial prepositions are
commonly used when individuals must determine how to get from one
location to the next, the spatial relationships between particular
locations, and the orientations and associations that comprise
critical features of objects in environments. A considerable amount
of experimental research has examined the use of such prepositions,
in combination with general expectations that comprehenders have for
orientations and perspectives (e.g., Carlson-Radvansky & Radvansky,
1996; Jackendoff & Landau, 1991; Landau & Jackendoff, 1993; Miller &
Johnson-Laird, 1976).
How do
comprehenders encode this information and combine it to construct a
representation with which they might navigate environments, consider
novel relationships, or simply think about described locations?
Psycholinguistics, the psychological study of language use, has
focused more generally on how any type of linguistic representation
may be constructed. This general interest proves directly
applicable for considering how individuals build representations for
space. More specifically, work on text comprehension has assessed
how language experiences influence representations, and the use of
those representations to solve problems, in the domain of spatial
cognition. In this section, we will consider three general topics
that focus on how humans update their representations of space based
on linguistic experience. These include the processes involved in
building a spatial representation, the circumstances associated with
resolving inconsistencies in such a representation, and the role of
individual differences. We note that while this research is from
the domain of text processing, the cognitive activities described
are likely generalizable to a variety of discourse, and hence
spatial, experiences.
The
first issue to consider involves the ways in which comprehenders
initiate and update their spatial representations. When we first
receive some spatial information, whether it is based on a verbal
description from a friend, a text or graphic description from a AAA
travel guide, or even first-person movement through some
environment, we may attempt to build mental structures that
represent that information in memory. The goal of building such
memory, of course, is to have some stored representation that can be
retrieved at a later time point. Additionally, such structures
should be updatable as novel information is encountered. Within
psycholinguistics, one hypothesis as to how such structures are
constructed (and potentially updated) comes from work on the gradual
construction of memory. The structure-building hypothesis (Gernsbacher,
1990) contends that as new information is encountered, comprehenders
build a new structure in memory to represent that information. With
each new event and description, one of two general processes can
occur. Either the existing representation can become more
elaborated (usually when the new information is directly relevant or
coherent with the previous information), or a completely new
substructure must be built for the new information (such as when a
new topic is introduced).
This
hypothesis is directly relevant for considering spatial processes.
Given that space is three-dimensional, yet language is
two-dimensional, language users often describe space in a linear way
(Levelt, 1989; Linde & Labov, 1975; Zwaan & Radvansky, 1998). This
fosters the building and updating of a representation in line with
the structure-building framework. Each piece of information (e.g.,
providing directions: "First, take University Avenue to Central.
Then turn left near the supermarket.") is encoded with respect
to previous information, in the hopes of building a representative
mental structure (see also Haviland & Clark, 1974). Thus,
comprehenders update their spatial representations by incorporating
new information into existing structures.
The
framework we have just described makes sense for cases in which
information is coherent or in line with prior information. But what
happens when new information is inconsistent with what we already
know? For example, if we expect that a left turn will lead us to a
particular location, but in fact it does not, how do we contend with
such information? Work on mental models (e.g., Gentner & Stevens,
1983; Johnson-Laird, 1983; Kintsch & van Dijk, 1978) has addressed
some of the processes involved in such updating. Mental models are
complex, connected internal representations of external stimuli.
What differentiates work on mental models from general work on
memory traces has been the specific focus on mental models as (a)
representing information beyond that which has been personally
experienced or read and (b) mentally manipulable structures, in that
they can afford the construction of novel inferences and
information. To this first point, mental models encode
relationships that have not been explicitly detailed. For example,
if A > B, and B > C, we might generate the inference that A > C,
even though such information was not explicitly described. A mental
model facilitates such operations. Secondly, mental models can be
manipulated as, for example, if you were asked to consider what your
neighborhood might look like from an overhead or survey perspective,
as compared to your familiar route perspective. Mental models are
the representations we use to run such simulations, and they are
invaluable as a method for constructing comprehension (Barsalou,
1999; Kahneman & Tversky, 1982). We should note that the
structure-building framework relies on the notion of mental models,
and research has explicitly associated that framework with the
construction of mental models (e.g., Rapp & Taylor, 2004).
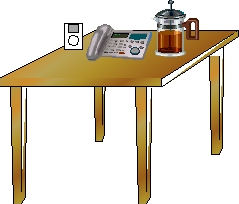 |
Figure 4. A spatial array on which a simple spatial description may
be based.
|
To
return to the second issue, what happens when new information is
inconsistent with the model we have built so far? For example,
consider the following spatial description: (1) The coffee pot is
to the right of the phone. (2) The iPod is to the left of the
phone. Now consider a final statement: (3) The coffee pot is to the
left of the iPod. This third statement cannot be true given the
prior information (see Figure 4). Ehrlich and Johnson-Laird (1982)
addressed this notion of inconsistency, finding that participants
take longer to read outcomes that are inconsistent with their
expectations [also see deVega (1995) and Rapp and Taylor (2004) for
further discussion]. These longer reading times suggest that
readers are working to reconcile the apparent inconsistency. With
respect to the structure-building framework, readers must either
revise their previous model (to reflect that, perhaps, one of the
objects was not in the right place or perhaps there were two iPods
or coffee pots), or build an entirely new structure to account for
the discrepancy. The goal, of course, is to maintain coherence
between new and prior information (Kendeou et al., 2004).
When coherence cannot be maintained, previous representations may be
overwritten or ignored in favor of new, more valid mental models.
A
considerable amount of work on this issue of updating, both with
consistent and inconsistent information, has been derived from
research examining spatial descriptions in narratives (Bower &
Morrow, 1990). We describe this work specifically not because it
represents the only way psychologists have studied spatial cognition
during reading, but because it represents a rich field of research
that has examined how spatial updating directly impacts
comprehension (e.g., Bower & Rinck, 2001; Morrow, 1994; Morrow,
Greenspan, & Bower, 1987; Morrow, Bower, & Greenspan, 1989; Rinck & Bower, 1995;
Rinck, Williams, Bower, & Becker, 1996; Rinck, Hahnel, Bower, &
Glowalla, 1997; Rinck, Bower, & Wolf, 1998; Wilson, Rinck, McNamara,
Bower, & Morrow, 1993). In this work,
participants are often asked to memorize spatial layouts of
buildings (for example map, see Figure 5), including objects found
in each room. Following memorization, they read stories describing
characters moving through the layout. Their reading is occasionally
interrupted with probe word pairs naming objects in rooms from the
layout. The task is to determine whether the objects would be found
in the same room (i.e., were two objects located in a single room)
or different rooms. Intriguing effects are obtained when
participants are reading about a particular room but the probes name
objects located in other rooms; readers take longer to make
decisions about objects in rooms far from the current focus (e.g.,
the protagonist's current location) and out of sequence, as compared
to rooms closer to that focus. Indeed, far and near are defined
here not by Euclidean distance, but with respect to the functional
organization of the rooms such as walls and doorways.
|
Figure 5. An example building layout, including
rooms and objects within rooms learned prior to reading about a
protagonist moving through the building. Such map stimuli were used
by Morrow et al. (1987) and Rapp et al. (in press).
|
Thus, readers' representations of locations are influenced directly
by the descriptions they read (i.e., the narratives), the knowledge
they already possess (i.e., their memory for the studied map), and
the current focus in the story (Rapp, Klug, & Taylor, in press). This
set of results suggests that readers update their spatial
representations on a moment-by-moment basis, reflecting the dynamic
situations described in narratives. The activation of spatial
information during reading likely fluctuates, in this way, over the
course of a narrative (e.g., Kendeou & van den Broek, 2005; van
den Broek, Risden, Fletcher, & Thurlow, 1996; van den Broek, Rapp, &
Kendeou, 2005),
potentially "out of sequence." So, updating is a process of these
fluctuating activations influencing, and being influenced by, what
people read (Rapp & van den Broek, 2005). Updating is the process
of comprehending linguistic input by relying upon the activation of
information in memory to facilitate coherent representations. These
processes occur as readers build models of space.
Of
course, not everyone can successfully update their memory and build
strong spatial representations. This leads to a third core issue in
the study of spatial updating through language - the role of
individual differences. Consider that individuals possess diverse
storehouses of prior knowledge, which as discussed earlier is
recruited in attempts to navigate environments and understand
space. For example, some readers may have more familiarity with the
Boston area, and thus a description of local Boston landmarks may be
more or less useful in understanding where to get the best ice cream
in the city. While many accounts of spatial cognition have focused
on individual differences in some inherent spatial ability (Allen,
2000; Gilmartin, 1986; Montello, Lovelace, Golledge, & Self, 1999;
Voyer, Voyer, & Bryden, 1995), just as important is the notion of whether individuals posses
prior knowledge that they can then apply to novel situations (van
den Broek et al., 2005). For example, if one is familiar with a
grid-like road layout, navigating New York's city layout would be
simple; prior knowledge about city grids can facilitate expectations
about how other grid-like city layouts work. However, knowledge about
that system is less useful in cities without such a layout (e.g.,
Boston). So, individual differences in spatial updating can be a
function of prior knowledge and experiences with spatial
situations.
Additionally, individual differences need not just be a function of
stored knowledge or inherited attributes, but also the particular
circumstances surrounding a spatial experience. Related to this
issue is a finding directly relevant to readers' general updating
processes. Work has shown that readers do not spontaneously update
their spatial models; instead, updating must be set as a specific
task goal (Hakala, 1999; Morrow, 1994; Rich & Taylor, 2000). This
suggests that spatial updating is not a privileged, automatic
process while reading. Only when circumstances demand such
updating, or individuals are specifically tracking the spatial
constraints of a situation, are they likely to update their spatial
models. Current work on spatial updating attempts to determine the
linguistic cues that foster such updating, as well as how
expectations may influence such processes (e.g., Rapp & Taylor,
2004). Overall, though, individual differences beyond proposed
genetic bases for spatial cognition tend to exert a powerful role on
spatial comprehension.
Obtaining spatial information through language is obviously a
uniquely human endeavor. Human comprehenders rely on language for
building and furthering an understanding of their environment.
Indeed, comprehension is a function not only of direct environmental
experience, but also indirect descriptions provided by others
through writing, speech, and gesture. Just as one might build any
physical structure, the mental processes involved in spatial
comprehension entail the building of a mental structure that houses
information about experienced and described environments. This
research suggests that the processes that underlie spatial
cognition, that of constructing a representation and updating that
representation, seem to a degree invariant across a variety of
discourse experiences.
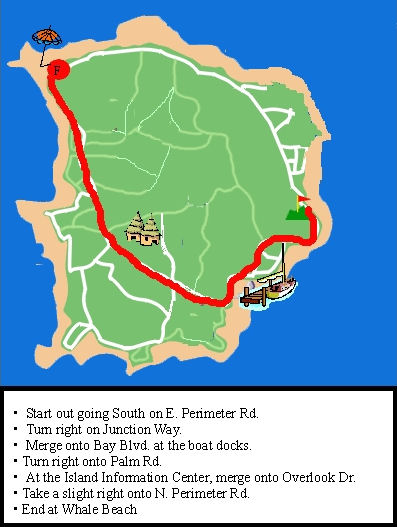 |
Figure 6. An example of
possible directions provided by internet direction/map sites.
|
While
psycholinguistics investigates the ways in which language influences
spatial processing, many everyday experiences involve more than just
the presentation of text in the service of learning about some
environment. One trip to Mapquest.com or Yahoo Maps on the internet
illustrates the common practice of combining spatial descriptions in
text format with pictorial maps (see Figure 6). People mentally
organize geographic space similarly whether they anticipate having
to verbally or graphically reproduce the environment (Taylor &
Tversky, 1992a), and they can make spatial inferences whether having
learned the information from maps or from descriptions (Taylor & Tversky, 1992b). Yet, these two symbolic information sources differ
in their inherent ability to present different information types.
Maps naturally convey specific spatial information, both relative
and absolute. Language naturally relates identity information
(through labels) and organizational, including sequencing,
information. Thus, maps combined with spatial descriptions (or vice
versa) maximize the potential for conveying spatial information.
In fact, it is generally the case (Cohen et
al., 1998; Wauchope, 1996) that a dual-mode
verbal and graphic representation is preferable to a single mode
presentation, as it may improve speed and accuracy of extracting
information. Considerable work by Mayer and colleagues (Mayer,
2001, 2003; Mayer & Sims, 1994) has demonstrated that combinations
of media formats (e.g., text and pictures) may facilitate learning.
Analogously, such combinations may assist in the construction and
use of reliable spatial representations. The caveat to this is that
such combinations should not tax cognitive load (Mayer, Heiser, &
Lonn, 2001), should not overwhelm perceptual processing (Tversky,
Morrison, & Betrancourt, 2002), should be appropriate to the information content (Brunye,
Taylor, & Rapp, 2005), and should match one's conceptualization of the
information (Tversky et al., 2002).
An important consideration for much of the topics under discussion
in this chapter is the developmental trajectories that might
influence spatial cognition. We have focused particularly on some
of the types of updating that are involved in using spatial
representations. However, the ways in which individuals at
different ages might show more or less proficiency in spatial
updating are only beginning to be considered (Newcombe & Sluzenski,
2004). For example, Uttal, Fisher, and Taylor (2006)
illustrated how maps and spatial descriptions may be symbiotically
combined to enhance mental representations. In their study,
8-year-olds, 10-year-olds and adults learned the layout of a
six-room space, either from a verbal description or from a map, and
were then tested on their configural and relative location memory.
For the types of information tested, participants who learned from
the map performed better than those who learned from the
description. For those who learned from descriptions, while the
ten-year-olds performed nearly as well as did the adults,
eight-year-olds' mental models differed substantially. The
8-year-olds retained sequential information but did not infer the
configural shape from the description. A second experiment showed
the advantage in updating conferred by seeing a graphic with the
description. In this study, participants of all ages first viewed a
schematic map of the spatial layout. This schematic provided only
layout information, omitting labeling and specifics about
locations. Seeing the schematic facilitated 8-year-old's use of the
description and improved their ability
to infer relative locations that had not been specifically
described. As this study demonstrates, insight into the development
of spatial cognition can inform our understanding of the mechanisms
that drive spatial updating.
Navigation through environments
requires the capacity to encode information about what we
experience. However, successful navigation, like successful problem
solving, requires that those spatial representations be updated to
incorporate new or changed information and to be used "out of
sequence." Our opening example presented one common case for which
this is important - revising our path to work or school to
accommodate construction detours. While we must rely on memory to
decide which streets we might take to get to work, we must also go
beyond those experience-driven, route based perspectives to consider
alternative paths to work when, say, a road is closed. Early
research demonstrated that non-human animals engage in similar
activities, determining shortcuts to retrieve a desired reward.
Thus, across species, the ability to successfully build spatial
representations is important for everyday survival.
Unlike those non-human animals,
though, human spatial cognition represents a more complex set of
processes and mechanisms that is designed to handle a diverse set of
experiences, cues, and symbols. For example, symbolic
representations, conveyed through pictures and language, can be used
to build robust representations for spatial environments of many
types. In fact, individuals can build vivid spatial representations
for places they might never actually navigate, including both
real-world (e.g., learning about a geographic region by reading
travel guides) and fictional settings (e.g., becoming familiar with
Hogwart's Castle layout from reading the Harry Potter series).
Because humans gain information from multiple sources, the mental
representation they form has been described as a cognitive collage (Tversky,
1993).
In this chapter, we have described
how spatial representations are both constructed and updated based
on a plethora of stimuli-driven, goal-driven, and participant-driven
features. By understanding the mechanisms and influences on spatial
cognition, we determine the underlying processes that guide spatial
activity (e.g., memory updating, perspective taking, or problem
solving). Additionally, that information may prove useful in the
development of information delivery systems (e.g., GIS, on-line map
services, or travel guidebooks) for helping individuals quickly and
effectively build spatial representations (Albert & Golledge, 1998).
In that sense, this work may inform the design of effective
learning systems (Rapp, 2005; Rapp, Taylor, & Crane, 2003).
Understanding how we build spatial representations, through direct
experience and symbolic experiences, both in sequence and out of
sequence, proves critical for both basic and applied settings.
Albert, W.S., & Golledge, R.G. (1998). The use of spatial cognitive
abilities in geographic information systems: The map overlay
operation. Transactions in GIS, 3(1), 7-21.
Allen, G.L. (2000). Men and women, maps and minds: Cognitive bases
of sex-related differences in reading and interpreting maps. In S.
O'Nuallain (Ed.), Spatial cognition: Foundations and
applications (pp. 3-18). Amsterdam: John Benjamins.
Avraamides, M.N., Klatzky, R.L., Loomis, J.M., & Golledge, R.G.
(2004). Use of cognitive versus perceptual heading during imagined
locomotion depends on the response mode. Psychological Science,
15(6), 403-408.
Barsalou, LW. (1999). Perceptual symbol systems. Behavioral &
Brain Sciences, 22, 577-660.
Bennett, A.T.D. (1996). Do animals have cognitive maps? The
Journal of Experimental Biology, 1999, 219-224.
Bilge, A.R., & Taylor, H.A. (2006). Learning nested environment from
maps: Is spatial updating simultaneous? Unpublished data. Tufts
University.
Bower, G.H., & Morrow, D.G. (1990). Mental models in narrative
comprehension. Science, 247(4938), 44-48.
Bower, G.H., & Rinck, M. (2001). Selecting one among many referents
in spatial situation models. Journal of Experimental Psychology:
Learning, Memory, and Cognition, 27, 81-98.
van
den Broek, P., Rapp, D.N., & Kendeou, P. (2005). Integrating
memory-based and constructionist processes in accounts of reading
comprehension. Discourse Processes, 39, 299-316.
van
den Broek, P., Risden, K., Fletcher, C.R., & Thurlow, R. (1996). A
"landscape" view of reading: Fluctuating patterns of activation and
the construction of a stable memory representation. In B. K. Britton
& A. C. Graesser (Eds.), Models of understanding text (pp.
165-187). Hillsdale, NJ: Lawrence Erlbaum Associates.
Brunye, T.T., Taylor, H.A., & Rapp, D.N. (2005). Putting things
together: Memory mechanisms underlying the benefits of multimedia
learning. Paper presented at the 46th Annual Meeting of the
Psychonomic Society, Toronto, Ontario.
Carlson-Radvansky, L.A., & Radvansky, G.A. (1996). The influence of
functional relations on spatial term selection. Psychological
Science, 7(1), 56-60.
Cohen, P., Johnston, M., McGee, D., Oviatt, S.,
Clow, J., & Smith, I. (1998). The efficiency of multimodal
interaction: A case study. Paper presented at the International
Conference on Spoken Language Processes, Australia.
deVega, M. (1995). Backward updating of mental
models during continuous reading of narratives. Journal of
Experimental Psychology: Learning, Memory, and Cognition, 21,
373-385.
Diwadkar, V.A., & McNamara, T.P. (1997).
Viewpoint dependence in scene recognition. Psychological Science,
8(4), 302-307.
Easton, R.D., & Sholl, M.J. (1995). Object-array
structure, frames of reference, and retrieval of spatial knowledge.
Journal of Experimental Psychology: Learning, Memory, and
Cognition, 21(2), 483-500.
Ehrlich, K., & Johnson-Laird, P.N. (1982).
Spatial descriptions and referential continuity. Journal of
Verbal Learning and Verbal Behavior, 21(3), 296-306.
Farrell, M.J., & Robertson, I.H. (1998). Mental
rotation and automatic updating of body-centered spatial relations.
Journal of Experimental Psychology: Learning, Memory, and
Cognition, 24(1), 227-233.
Foo, P., Wareen, W.H., Duchon, A., & Tarr, M.J.
(2005). Do humans integrate routes into a cognitive map? Map-
versus landmark-based navigation of novel shortcuts. Journal of
Experimental Psychology: Learning, Memory, and Cognition, 31(2),
195-215.
Gallistel, C.R. (1990). The organization of
learning. Cambridge, MA: M.I.T. Press.
Gattis, M. (2001). Spatial schemas and
abstract thought. Cambridge, MA: MIT Press.
Gauvain, M. (2001). Cultural tools, social
interaction and the development of thinking. Human Development,
44, 126-143.
Gentner, D., & Stevens, A.L. (1983). Mental
models. Hillsdale, NJ: Lawrence Erlbaum Associates.
Gernsbacher, M.A. (1990). Language
comprehension as structure building. Hillsdale, NJ: Lawrence
Erlbaum Associates.
Gilmartin, P. (1986). Maps, mental imagery, and
gender in the recall of geographic information. The American
Cartographer, 13, 335-344.
Givon, T. (1992). The grammar of referential
coherence as mental processing instructions. Linguistics. Special
topic: From grammar to discourse, 30(1), 5-55.
Glasgow, J., Narayanan, N.H., & Chandrasekaran,
B. (Eds.). (1995). Diagrammatic reasoning: Cognitive and
computational perspectives. Menlo Park, CA: AAAI Press.
Golledge, R.G., & Spector, N.A. (1978).
Comprehending the urban environment: Theory and practice.
Geographical Analysis, 14, 305-325.
Gouteux, S., & Spelke, E.S. (2001). Children's
use of geometry and landmarks to reorient in an open space.
Cognition, 81, 119-148.
Hakala, C. (1999). Accessibility of spatial
information in a situation model. Discourse Processes, 27,
261-280.
Haviland, S.E., & Clark, H.H. (1974). What's
new? Acquiring new information as a process in comprehension.
Journal of Verbal Learning and Verbal Behavior, 13(5), 512-521.
Hermer, L., & Spelke, E. (1994). A geometric
process for spatial representation in young children. Nature,
370, 57-59.
Jackendoff, R., & Landau, B. (1991). Spatial
language and spatial cognition. In D. J. Napoli & J. A. Kegl (Eds.),
Bridges between psychology and linguistics: A swarthmore
festschrift for lila gleitman (pp. 145-169). Hillsdale, NJ:
Lawrence Erlbaum Associates.
Johnson-Laird, P.N. (1983). Mental models:
Toward a cognitive science of language, inference, and consciousness.
Cambridge, MA: Harvard University Press.
Kahneman, D., & Tversky, A. (1982). The
simulation heuristic. In D. Kahneman, P. Slovic, & A. Tversky
(Eds.), Judgment under uncertainty: Heuristics and biases
(pp. 201-208). New York: Cambridge University Press.
Kendeou, P., Rapp, D.N., & van den Broek, P.
(2004). The influence of readers' prior knowledge on text
comprehension and learning from text. In R. Nata (Ed.), Progress
in education (pp. 189-209). New York: Nova Science Publishers,
Inc.
Kendeou, P., & van den Broek, P. (2005). The
effects of reader's misconceptions on comprehension of scientific
text. Journal of Educational Psychology, 97, 235-245.
Kintsch, W., & van Dijk, T.A. (1978). Toward a
model of text comprehension and production. Psychological Review,
85(5), 363-394.
Kuipers, B. (2000). The spatial semantic
hierarchy. Artificial Intelligence, 119, 191-233.
Landau, B., & Jackendoff, R. (1993). "What" and
"where" in spatial language and spatial cognition. Behavioral &
Brain Sciences, 16(2), 217-264.
Leiser, D., & Zilbershatz, A. (1989). The traveller: A computational
model of spatial network learning. Environment and Behavior, 21,
435-463.
Levelt, W.J.M. (1989). Speaking: From intention to articulation.
Cambridge, MA: MIT Press.
Linde,
C., & Labov, W. (1975). Spatial networks as a site for the study of
language and thought. Language, 51(4), 924-939.
MacEachren, A.M. (1994). Some truth with maps: A primer on
symbolization and design. Washington, D.C.: Assocation of
American Geographers.
MacEachren, A.M. (1995). How maps work: Representation,
visualization, and design. New York: Guilford Press.
May, M. (2004). Imaginal perspective switches in remembered
environments: Transformation versus interference accounts.
Cognitive Psychology, 48(2), 163-206.
Mayer, R.E. (2001). Multimedia learning. New York: Cambridge
University Press.
Mayer, R.E. (2003). The promise of multimedia learning: Using the
same instruction design methods across different media. Learning
and Instruction, 13(2), 125-139.
Mayer, R.E., Heiser, J., & Lonn, S. (2001). Cognitive constraints on
multimedia learning: When presenting more material results in less
understanding. Journal of Educational Psychology, 93(1),
187-198.
Mayer, R.E., & Sims, V.K. (1994). For whom is a picture worth a
thousand words? Extensions of a dual-coding theory of multimedia
learning. Journal of Educational Psychology, 86(3), 389-401.
McNamara, T.P., Hardy, J.K., & Hirtle, S.C. (1989). Subjective
hierarchies in spatial memory. Journal of Experimental
Psychology: Learning, Memory, and Cognition, 15, 211-227.
McNamara, T.P., Rump, B., & Werner, S. (2003). Egocentric and
geocentric frames of reference in memory of large-scale space.
Psychonomic Bulletin & Review, 10(3), 589-595.
McNamara, T. P., & Shelton, A. L. (2003). Cognitive maps and the
hippocampus. Trends in Cognitive Science, 7(8), 333-335.
Miller, G.A., & Johnson-Laird, P.N. (1976). Language and
perception. Oxford, England: Harvard University Press.
Montello, D.R., Lovelace, K., Golledge, R.G., & Self, C. (1999).
Sex-related differences and similarities in geographic and
environmental spatial abilities. Annals of the Association of
American Geographers, 89, 515-534.
Morrow, D.G. (1994). Spatial models created from text. In H. van
Oostendorp & R. A. Zwaan (Eds.), Naturalistic text comprehension
(pp. 57-78). Norwood, NJ: Ablex Publishing Corporation.
Morrow, D.G., Bower, G.H., & Greenspan, S.L. (1989). Updating
situation models during narrative comprehension. Journal of
Memory and Language, 28, 292-312.
Morrow, D.G., Greenspan, S.L., & Bower, G.H. (1987). Accessibility
and situation model narrative comprehension. Journal of Memory
and Language, 26, 165-187.
Mou,
W., & McNamara, T.P. (2002). Intrinsic frames of reference in
spatial memory. Journal of Experimental Psychology: Learning,
Memory, and Cognition, 28(1), 162-170.
Newcombe, N.S., & Sluzenski, J. (2004). Knowing where things are in
the second year of life: Implications for hippocampal development.
Journal of Cognitive Neuroscience. Special Issue on
Developmental Cognitive Neuroscience, 16(8), 1443-1451.
O'Keefe, J., & Nadel, L. (1978). The
hippocampus as a cognitive map. Oxford: Oxford University Press.
Plumert, J. M., Ewert, K., & Spear, S.J. (1995). The early
development of children's communication about nested spatial
relations. Child Development, 66, 959-969.
Presson, C. C., & Montello, D. R. (1994). Updating after rotational
and translational body movement: Coordinate structure of
perspective space. Perception, 23(12), 1447-1455.
Rapp,
D.N. (2005). Mental models: Theoretical issues for visualizations
in science education. In J. K. Gilbert (Ed.), Visualization in
science education (pp. 43-60). The Netherlands: Springer.
Rapp,
D.N., Klug, J.L., & Taylor, H.A. (in press). Expectation-driven
effects on spatial mental model use during reading. Memory &
Cognition.
Rapp,
D.N., & Taylor, H.A. (2004). Interactive dimensions in the
construction of mental representastions for text. Journal of
Experimental Psychology: Learning, Memory, and Cognition, 30,
988-1001.
Rapp,
D.N., Taylor, H.A., & Crane, G. (2003). The impact of digital
libraries on cognitive processes: Psychological issues of
hypermedia. Computers in Human Behavior, 19, 609-628.
Rapp,
D.N., & van den Broek, P. (2005). Dynamic text comprehension: An
integrative view of reading. Current Directions in Psychological
Science, 14, 276-279.
Rich,
S.S., & Taylor, H.A. (2000). Not all narrative shifts function
equally. Memory & Rieser, J.J. (1989). Access to knowledge of
spatial structure at novel points of observation. Journal of
Experimental Psychology: Learning, Memory, and Cognition, 15(6),
1157-1165.
Rieser, J.J., Garing, A.E., & Young, M.F. (1994). Imagery, action,
and young children's spatial orientation: It's not being there that
counts, it's what one has in mind. Child Development, 65(5),
1262-1278.
Rinck,
M., & Bower, G.H. (1995). Anaphora resolution and the focus of
attention in situation models. Journal of Memory and Language, 34,
110-131.
Rinck,
M., Bower, G.H., & Wolf, K. (1998). Distance effects in surface
structures and situation models. Scientific Studies of Reading, 2,
221-246.
Rinck,
M., Hahnel, A., Bower, G.H., & Glowalla, U. (1997). The metrics of
spatial situation models. Journal of Experimental Psychology: Learning, Memory, and Cognition, 23, 622-637.
Rinck,
M., Williams, P., Bower, G.H., & Becker, E.S. (1996). Spatial
situation models and narrative understanding: Some generalizations
and extensions. Discourse Processes, 21, 23-55.
Shelton, A. ., & McNamara, T.P. (1997). Multiple views of
spatial memory. Psychonomic Bulletin & Review, 4(1), 102-106.
Shelton, A.L., & McNamara, T.P. (2001a). Systems of spatial
refererence in human memory. Cognitive Psychology, 43(4),
274-310.
Shelton, A.L., & McNamara, T.P. (2001b). Visual memories from
nonvisual experiences. Psychological Science, 12(4), 343-347.
Sholl,
M.J. (1987). Cognitive maps as orienting schemata. Journal of
Experimental Psychology: Learning, Memory, and Cognition, 13,
615-628.
Sholl,
M.J. (2000). The functional separability of self-reference and
object-to-object systems in spatial memory. In S. O'Nuallain (Ed.),
Spatial cognition: Foundations and applications (pp. 45-67).
Amsterdam: John Benjamins.
Sholl,
M.J., & Nolin, T.L. (1997). Orientation specificity in
representation of place. Journal of Experimental Psychology: Learning, Memory, and Cognition, 23(6), 1494-1507.
Taylor, H.A., Naylor, S. J., & Chechile, N.A. (1999). Goal-directed
influences on the representation of spatial perspective. Memory
and Cognition, 27(2), 309-319.
Taylor, H.A., & Tversky, B. (1992a). Descriptions and depictions of
environments. Memory & Cognition, 20, 483-496.
Taylor, H. A., & Tversky, B. (1992b). Spatial mental models derived
from survey and route descriptions. Journal of Memory and
Language, 31, 261-282.
Taylor, H.A., & Tversky, B. (1996). Perspective in spatial
descriptions. Journal of Memory and Language, 35, 371-391.
Thorndyke, P.W., & Hayes-Roth, B. (1982). Differences in spatial
knowledge acquired from maps and navigation. Cognitive
Psychology, 14, 560-589.
Tolman, E.C. (1948). Cognitive maps in rats and men.
Psychological Review, 55, 189-208.
Tolman, E.C., & Honzik, C.H. (1930). Insight in rats. University
of California Publications in Psychology, 4, 215-232.
Tversky, B. (1993). Cognitive maps, cognitive collages, and spatial
mental models. In A.U. Frank & I. Campari (Eds.), Spatial
information theory: A theoretical basis for GIS (pp. 14-24).
Berlin: Springer-Verlag.
Tversky, B., Morrison, J.B., & Betrancourt, M. (2002).
Animation: Can it facilitate? International Journal of
Human-Computer Studies, 57(4), 247-262.
Uttal,
D.H. (2000). Seeing the big picture: Map use and the development of
spatial cognition. Developmental Science, 3, 247-286.
Uttal,
D.H., Fisher, J.A., & Taylor, H.A. (2006). Words and maps: Developmental changes in mental models of spatial information.
Developmental Science,
9(2), 221-235.
Voyer,
D., Voyer, S., & Bryden, M.P. (1995). Magnitude of sex differences
in spatial abilities: A meta-analysis and consideration of critical
variables. Psychological Bulletin, 117, 250-270.
Waller, D., Loomis, J.M., & Haun, D.B.M. (2004). Body-based senses
enhance knowledge of directions in large-scale environments.
Psychonomic Bulletin & Review, 11(1), 157-163.
Wang,
R.F. (2004). Between reality and imagination: When is spatial
updating automatic? Perception & Psychophysics, 66(1), 68-76.
Wang,
R.F., & Brockmole, J.R. (2003a). Human navigation in nested
environments. Journal of Experimental Psychology: Learning,
Memory, and Cognition, 29(3), 398-404.
Wang,
R.F., & Brockmole, J.R. (2003b). Simultaneous spatial updating in
nested environments. Psychonomic Bulletin & Review, 10(4),
981-986.
Wang,
R.F., & Spelke, E.S. (2000). Updating egocentric representations in
human navigation. Cognition, 77, 215-250.
Wang, R.F., & Spelke, E.S. (2002). Human spatial
representation: Insights from animals. Trends in Cognitive
Sciences, 6, 376-382.
Wauchope, K. (1996). Multimodal interaction with a map-based
simulation system (Technical Report No. AIC-96-019). Washington,
D.C.: Naval Research Laboratory.
Wilson, S.G., Rinck, M., McNamara, T. P., Bower, G.H.,, & Morrow, D.
G. (1993). Mental models and narrative comprehension: Some
qualifications. Journal of Memory and Language, 32, 141-154.
Zwaan,
R.A., & Radvansky, G.A. (1998). Situation models in language
comprehension and memory. Psychological Bulletin, 123(2),
162-185.
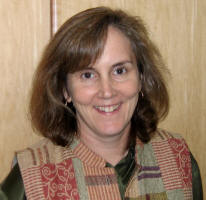
© 2006 All
copyrights for the individual chapters are retained by the authors.
All other material in this book is copyrighted by the editor, unless
noted otherwise. If there has been an error with regards to
unacknowledged copyrighted material, please contact the editor
immediately so that this can be corrected. Permissions for using
material in this book should be sent to the editor.