Amniote Evolution
Onto
the land: Tetrapod origins. All extant tetrapods (i.e., amphibians,
reptiles, birds, and mammals) originally derived from water-dwelling amphibians.
Their amphibial ancestors first appear somewhere in the Devonian period
of the Paleozoic era (Norman, 1994). The Paleozoic amphibians laid
jelly-covered eggs in water, as do their modern counterparts. However,
there are many differences between the Paleozoic and modern amphibians.
Generally, the Paleozoic amphibians were rather large (0.6 - 1 meter long)
and, although they possessed legs, were primarily water-based and fed mainly
on bony fish (Norman, 1994).
The reproductive strategy of amphibians
was not a suitable means of reproduction for those tetrapods who broke
with a water-based existence. Moving from shorelines to farther
inland, these forms began to lay
their eggs on land, and embryos were protected
by membranes (i.e., amnion) and hard shells. With the innovation
of the shelled egg, these organisms were closer to fully integrating into
land-based lifestyles. These were the earliest reptiles, whose modern descendants
are included in the larger classification of the amniotes.
The earliest amniotes were the Captorhinida,
which eventually gave rise to modern amniotes (reptiles, birds, and mammals).
The ancient reptilian group the Captorhinida derived early in the
Carboniferous (360-286 Million Years Ago; MYA) from the anthracosaurs,
a group of Paleozoic amphibians (Carroll, 1988).
By the end of the Carboniferous, amniotes
could be categorized into three groups on the basis of skull anatomy.
There are openings in the dermal skull roof behind the orbits, whose pattern
is used for classifying the ancestral amniotes. The earliest amniotes,
including the Captorhinida, had no such opening, and they are called Anapsida.
Turtles are often included in this group since their skull is entirely
covered with bone. The first group which diverged from the early
anapsids are the Synapsida (one temporal opening), which ultimately
evolved into mammals. The second group which separated from the basal
anapsids were the Diapsida (two openings), which evolved into reptiles
(including dinosaurs) and birds.
Birds. The diapsids eventually gave rise to
the dinosaurs, modern reptiles, and birds. Diapsids are further
divided into two major groups: the Lepidosauromorphs and the Archosauromorphs.
The former group includes sphenondontids, lizards, and snakes, whereas
the latter includes archosaurs, such as dinosaurs and crocodiles.
The earliest birds are phylogenetically close to the archosaurs, but the
precise origin and evolution of birds is still the matter of some debate.
The first true birds appear sometime in the late Jurassic or perhaps earlier
(for details see the section Dinosaurs
Among Us?, this chapter).
Major radiations of birds occurred during
the Cretaceous (135-65 MYA) and the Tertiary period of the Cenozoic era
(65-1.9 MYA). In the 85 million year span between the Jurassic
and the beginning of the Cenozoic, two major groups of Cretaceous birds
arose, the Enantiornithes and the Ornithurines. The
Enantiornithes ("opposite birds") were so named because of their metatarsals
being fused proximal to distal, which is opposite that of modern birds
(Feduccia, 1996). The Ornithurines eventually led to currently existing
modern forms, along with extinct loon-like Hesperornithiformes (toothed
birds, mostly flightless swimmers) and tern-like Ichthyornithiformes (toothed
flying birds which probably fed on fish). From existing evidence,
it appears none of the toothed birds survived the Cretaceous extinction.
There is some scattered evidence that the ancestors of modern shorebirds
may have extended back into the late Cretaceous and survived the massive
extinctions in which the dinosaurs perished.
Most modern birds have ancestors which
appeared by the end of the Miocene (5.5 MYA). The diversification
of birds after the Cretaceous extinctions was very rapid, with the ornithurine
birds diverging into
almost all the present orders within 5-10 million
years. Modern bird orders appear in the late Paleocene and by the
Oligocene most of the orders of birds recognized today were in existence.
By the Miocene the dominant land birds had become the passerines.
However, none of those actual Miocene species during that period survive
today. All modern species probably have evolved only in the last
1-2 million years. Data from studies of the accumulation of point
mutations causing amino acid substitution in proteins between all 27 orders
of birds indicate a relatively modest change between species. This
conservation of genetic sequences indicates a rapid divergence of species
in only the past million years or so (Feduccia, 1996).
Birds are the most successful of the
terrestrial vertebrates, found on every continent and in almost every ecological
niche. Birds are a diverse group, with over 9,000 species, compared
to the amphibians (3,000 species), reptiles (6,000), and mammals (4,100);
(Chatterjee, 1997). There are 27 to 28 orders of modern birds that
can be classified into two superorders, the Palaeognathae and Neognathae.
The Paleaognathae include the emu, ostrich, rhea, cassowary, tinamous,
and kiwi; most of these forms have lost the ability to fly. The Neognathae
encompass all other orders of birds and is divisible into the passerine
(perching) and non-passerine subgroups. The passerines
incude zebra finches, swallows, and sparrows; the non-passerines include
owls, chickens, quail, and pigeons.
Mammals. Mammals evolved from Mesozoic synapsid
reptiles, which included the pelycosaurs and therapsids. Mammals
diverged from this reptilian group around the late Triassic / early Jurassic,
about 200 million years ago.
The Mammalia consist of three major divisions, representing three branches
which derived from the therapsids (mammal-like reptiles). The three
present classifications of mammals, the monotremes, marsupials,
and placentals, arose at an early stage and thus have evolved independently
during that time. The monotremes are egg-laying forms, whose
only modern representatives are the duck-bill platypus (Ornithorhynchus)
and the spiny ant-eater, or echidna (Tachyglossus and Zaglossus).
The marsupials have eggs but hatch them inside the body and bear
the young in an embryonic condition. The young then continue their development
typically in an abdominal pouch, feeding on the mother’s milk. The placentals make up the third classification and includes a huge
variety of organisms inhabiting sea (e.g., dolphins, whales), air (e.g.,
bats), and land (e.g., elephants, primates, horses). The earliest
placental mammals were insectivores. Sometime in the Mesozoic, they
diversified into several lines of descent, one of which was the Lipotyphla,
who continue to the present day in the hedgehogs, shrews, and moles.
From the Lipotyphla there derived a branch called the Menotyphla whose
living members are the tree and elephant shrews. Three branches at different
points in evolutionary history became the Dermoptera (flying lemurs; Galeopithecus
and Galeopterus), the Chiroptera (bats), and the Primates (Walls,
1942). Given the early divergence of the monotremes and placentals,
caution is warranted when looking to the egg-laying mammals (e.g., echidna
or platypus) as a kind of ancestral link between reptiles and modern placental
mammals.
Amniote
Brains
Many similarities exist in the overall
organization of modern amniote brains, and in the basic organization of
their visual systems. Our knowledge of the brains of ancestral
amniote forms is limited to what can be derived from fossil skulls.
Observations of the cranial structure provide gross morphological measures,
and endocasts can even provide some surface detail. From such data
tentative guesses about the olfactory bulbs, forebrain structure, optic
lobes, and cerebellar development can be made. Considering the relative
size and development of these structures can help somewhat in constructing
what kind of lifestyle the organism exhibited. This can be considered
with environmental and geographical factors probably existing at the time,
along with estimated predator/prey ratios, et cetera, to construct
a picture of how these long extinct organisms lived. Most extra-retinal
structures relevant to discussions of avian visual evolution are found
in the midbrain and forebrain regions.
Tectum. The tectum of the mesencephalon is
an important visual structure in the amniote visual system. The tectum receives topographic, highly organized projections from visual,
auditory, and somatosensory systems. The "maps" formed from this
input are in good register with each other, allowing the organism a well-formed
representation of its sensory space. The organization of this structure
also supports the coordination of behaviors elicited by visual, auditory,
or somatosensory stimuli in the sensory space. The optic lobe,
or optic tectum, plays a prominent role in the visual behaviors
of modern reptiles and birds. Its importance in mammals has historically
been over-shadowed by research into the more prominent geniculo-striate
system. However, the importance of the mammalian optic tectum (i.e.,
superior colliculus) in visual behaviors is becoming better appreciated
with studies in cats and primates (Chalupa, 1977; Petersen et al, 1985,
Ungerleider & Pribam, 1977).
Dorsal
Thalamus & Pallium. Structures in the diencephalon and
telencephalon are also crucial to visual behavior in amniotes.
The major visual recipient area of the diencephalon in all vertebrates
is the dorsal thalamus, which is composed of several nuclei which
relay ascending sensory information to the telencephalon. Dorsal
thalamic nuclei in amniotes which receive ascending visual input from the
retina receive such input either directly (as in the thalamofugal
or lemnothalamic pathway) or indirectly via the tectum (as in the tectofugal or collothalamic pathway). The telencephalon
is divisible into both a dorsal and ventral component, the pallium
and subpallium, respectively. The pallium is divisible into
dorsal, lateral (olfactory or piriform), and medial (limbic) divisions.
The subpallium includes the basal ganglia and septum.
Common to amniote forebrain organization
is that ascending sensory information is relayed through dorsal thalamic
nuclei to parts of both the pallium and subpallium. The pallial
areas tend to be specialized into discrete regions in receipt of afferents
from one particular sensory modality. In non-mammalian amniotes,
the dorsal pallium contains dorsal cortex. In birds, a rostral expansion
of the dorsal pallium which receives visual input from the lemnothalamic
system has been termed the visual wulst. The dorsal and lateral
pallia in mammals have undergone a spectacular expansion, developing into
the laminar isocortex. The six-layered cortex, with its marked
sulci and gyri, is often considered the defining characteristic of mammalian
brains, and was once considered a pre-requisite for true higher-level sensory
and cognitive activities. Subsequent research into the cognitive
and sensory capacities of birds and other organisms has discredited this
idea. The differences, however, between the avian/reptilian dorsal
ventricular ridge and mammalian isocortex are instructive in both the
attempt to reconstruct visual system evolution and understand visual processing
in these very different brains.
DVR
and Cortex
The dorsal ventricular ridge (DVR) is a pallial
structure unique to the non-mammalian amniotes (reptiles and
birds).
The telencephalon of different vertebrate forms
start similarly.
A vesicle at the rostral end of the neural tube is the foundation of the
telencephalon in all vertebrates. Subsequent development after this
formation differs among the amniotes. Reptiles and birds exhibit
the formation of the DVR, which protrudes into the lateral ventricle
and forms the lateral walls of the telencephalon. The DVR can be further
divided into anterior (ADVR) and basal portions (BDVR; called archistriatum in birds). In mammals, the floors of the hemispheres
protrude into the lateral ventricles to form the basal ganglia, while the
telencephalic roof expands and forms the distinctive lamination of the
isocortex (Ulinski, 1983).
Type
I and Type II DVR. The DVR is divisible into two basic
patterns (Type I and Type II) based on the distribution of neurons.
The first pattern is called Type I DVR, or a corticoid band-core
arrangement (Butler, 1980). Type I DVR demonstrates three relatively
distinct zones, defined by the cytology and distribution of neurons in
the ADVR (Ulinski, 1983). These zones have the following characteristics:
-
Dorsal Zone: relatively cell-poor zone with
a cluster of juxtaependymal neurons whose dendrites extend concentrically
with the ventricular surface
-
Second Zone: relatively larger numbers of
stellate neurons which form clusters, with the neurons' somata touching
-
Ventral Zone: consists of stellate neurons
with both scattered individual neurons and occasional clusters present
The stellate neurons of the second and third
layers can either be spiny or spine-poor. These neurons have a radial
orientation which enables them to form interconnections with each other.
This first pattern of DVR organization is found in snakes, tuatara (Sphenodon),
turtles, and most lizards.
In birds and crocodiles a second type
of organization is seen, called Type II DVR, or a nuclear arrangement.
Type II DVR does not possss a distinct periventricular cell cluster zone
in ADVR. In addition there are isolated neurons as well as prominent
neuronal clusters scattered throughout the forebrain in Type II DVR (Ulinski,
1983). Other characteristics include:
-
Dorsal region: juxtaependymal neurons with
dendrites concentric to the ventricular surface
-
Individual and clustered neurons are typically
spiny with stellate-shaped dendritic fields possessing variable numbers
of spines
In Alligator there is a tendency for clusters
to form a periventricular zone within the dorsomedial area but not in other
areas as in Type I reptiles. In birds, clusters of neurons
also occur throughout the ADVR. Several distinct types of neurons
have been recognized in different avian species, but most neurons can be
characterized as having stellate-shaped dendritic trees with varying numbers
of spines. In addition, there are neurons with radially oriented
connections seen in several sensory areas of birds (e.g., ectostriatum
to peri-ectostriatal belt; interconnections of Field L areas); (Ulinski,
1983).
Despite differences in DVR organization,
common to all reptiles and birds is the basic connectivity of the ADVR
and BDVR. Ulinski (1983) suggested that the basic organization
of the DVR is to serve as a linkage between sensory inputs and motor
outputs; an interface between sensory and perceptual processing and
mechanisms which modulate behavior.
ADVR receives visual, auditory, and somatosensory information and sends
outputs to the BDVR and basal ganglia. It also appears that this
is the basic function of mammalian isocortex. One of the overriding
questions in comparative neurobiology is the relationship between the neural
groupings characteristic of DVR and the laminar arrangement of neurons
found in the mammalian isocortex. This debate usually centers around
the question of whether their is a homologous relationship between DVR
and cortex.
Comparing
DVR & mammalian isocortex. The six layered isocortex in mammals
may not be as different from the DVR of diapsid reptiles and birds as it
appears. The radial, outward migration of neuroblasts from the
ventricular surface of the telencephalon results in the basic six layered
pattern characteristic of mammalian isocortex. Isocortical
neurons include the pyramidal cells in the third
and fifth layers and several non-pyramidal varieties. Despite the
morphological differences between DVR and cortex, these areas may process
information similarly. The isocortex is in a similar processing position
to that of DVR, being an area which links sensory inputs and directing
motor responses (Ulinski, 1983). Anatomical connections indicate
that the nuclear groupings of DVR could be homologous to certain areas
of mammalian isocortex. With the possibilities of convergent evolution,
and alternative theories of cortical evolution, however, DVR / isocortex
homology is still a debated theory (Butler & Hodos, 1996). There
is some evidence that a simple definition of cortex as a laminar arrangement
of cells and fibers may be too restrictive; some of the same populations
of cells present in cortex may be present in nonmammals, with similarities
in neural connections but arranged in a different fashion (Hodos, 1976).
The ADVR and mammalian
isocortex share similarities in the organization of their sensory circuits.
The ADVR has been compared to the mammalian isocortex based on the similarities
of sensory circuits between the nuclear groupings of non-mammalian amniote
brain and the distinctly laminar mammalian isocortex (Northcutt, 1981;
Karten & Shimizu, 1989; Reiner, 1993; Butler, 1994; Veenman et al.,
1995; Karten, 1997). Both the mammalian and avian systems are characterized
by major thalamic input to the telencephalon (e.g., layer IV in mammals;
the core ectostriatum in birds) which is then relayed to overlying areas
(layer IV projections to layers II and III in mammals; core ectostriatum
projections to peri-ectostriatal belt and overlying neostriatum in birds).
Additionally, in mammals, there are descending projections from layers
II and III to layers V and VI, from which output is directed to the tectum.
From the intermedial, lateral neostriatum in birds there are descending
projections to the archistriatum (Ritchie, 1979; Husband et al., 1995),
which in turn is a source of projections to the tectum (Zeier & Karten,
1971). The comparisons of cortical layers with the nuclear groupings
of birds noted above indicate a similar pattern of information processing
in amniote brains. These similarities could be due to homology; however,
they may also be unrelated to the condition of the ancestral amniote brain,
having arisen through other mechanisms. The source of the similarities
in patterns of amniote brain organization, therefore, remains unresolved.
Avian ADVR organization,
in conjunction with the outdated "-striatum" designation for avian forebrain
structures, may unnecessarily confuse comparisons of neuroanatomy and function
of amniote sensory systems. The ADVR in birds is organized into
cytoarchitectonically distinct cell groups along the dorsal-ventral axis:
a medially located neostriatum (including the ectostriatum) and a dorsally
located hyperstriatum. Several commonalities between the isocortex
and ADVR are apparent when considering the arrangement of sensory-recipient
areas in telencephalon. Both isocortex and ADVR have the cell groups
of their sensory circuits (i.e., cortical layers or ADVR nuclei) organized
perpendicular to the lateral ventricle. The afferent and efferent
connections, as well as their radial pattern of organization, indicate
that isocortex and ADVR share similar general principles of telencephalic
sensory processing. These similarities may have arisen with the ancestral
stem reptile which gave rise to modern amniotes. The stem reptiles
may have possessed nonlaminar groupings of functionally specialized neural
populations (Shimizu & Karten, 1993). However, anatomical
and functional similarities could have also evolved through convergence.
In other words. these similarites could be homoplastic
rather than homologous.
In any event, the neural systems of birds, reptiles, and mammals may have
much more in common than is evident
from the distinctly non-laminar appearance of sauropsid (aivan and reptile)
brains.
Brain
Evolution
Reconstructing the evolution of the
brain from the early tetrapods to modern forms poses several challenges.
The fossil record is incomplete and detailed endocasts are rare.
However, in those fossils and endocasts that have been discovered there
are some reasonable conclusions to be drawn about brain organization.
We can begin to reconstruct the rudiments of overall brain morphology from
ancestral to modern forms. By analyzing the similarities in modern
forms, from the crocodiles, turtles, birds, and mammals we can make assumptions
about the condition of the neural structures within the brain in their
amniote ancestors. Such reconstructions, however, must take into
account the long divergence of these forms from their common ancestor.
Forebrain Expansion. The pattern of the evolving brain has
been generally marked by changes in the ratio of midbrain and forebrain
structures, and in the expansion of the forebrain itself. These
transitions are seen in the evolution from stem reptiles to the "mammal-like"
reptiles (carnivorous pelycosaurs and therapsids)
to the ancient mammals. Forebrain expansion probably occurred
throughout the Jurassic and Cretaceous in all of the lineages leading to
modern birds, reptiles, and mammals. In a genus close to the stem
reptiles, Diadectes had a somewhat tubular and narrow midbrain and
forebrain, superficially resembling that of the lungfishes and amphibians
of today (Ulinski, 1983).
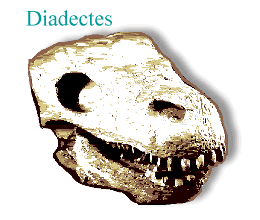
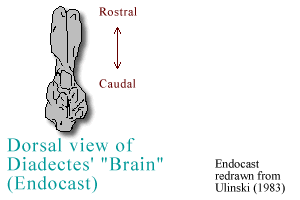
However, there is evidence modern amphibians
have undergone simplifications (e.g., in skeletal structure). These
present forms have also had a great deal of evolutionary time to evolve
and adapt to their particular niches; therefore caution is warranted when
considering them as models of the ancestral reptilian brain. Early
archosaurs, such as the pseudosuchians of the middle Triassic,
also exhibited narrow midbrains and forebrains. By the late Triassic,
however, the forebrain of archosaurs like Desmatosuchus had grown
to similar proportions of that seen in the Crocodilia (Hopson, 1979).
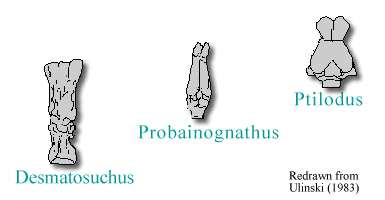
In the line of forms leading to mammals, pelycosaurs exhibited narrow midbrains and forebrains. Eventually,
a gradual increase in the relative size of brain versus body size is seen.
The therapsids, such as Probainognathus, possessed telencephalons
like elongate tubes. By the Mesozoic, the mammals show an expansion
of the forebrain, such as that in Ptilodus. Later placental
forms exhibit further widening and expansion of the forebrain, including
the sulcal patterns of the brain surface. One interpretation of the
aforementioned trends is that the forebrain expansion in the ancestors
of modern reptiles (and eventually birds) reflects the evolution of the
DVR, while the evolution of the forebrain in mammals reflects the expansion
of the basal ganglia and the uniquely mammalian isocortex (Ulinski, 1983).
Emergence of the DVR. The stem reptiles probably possessed
a Type I DVR. The basic DVR organization could probably be found
in the organisms which existed prior to their divergence into the lepidosaur
and archosaur lineages. Sensory system sophistication may be reflected
in the emergence of Type II DVR in birds. The Type II neuronal arrangement
involves both the migration of cells away from the ventricular surface
and a quantitative increase in the size of the ridge. This presumably
indicates the opportunity for more complex information processing capabilities
and perhaps more adaptability in behavioral responses to stimuli.
A type II lizard, Tupinambis, is an active arboreal forager which preys
on smaller vertebrates and insects using visual, auditory, and olfactory
senses. Tupinambis has the quantitatively largest DVR of all lizards
(Butler, 1980). In addition, the gecko, a member of the type I DVR
class, shares the foraging and arboreal habits of the iguanas and have
large DVRs which overlap with species in the lower ranges of the type II
reptiles (Butler, 1980). Increases in volume and a trend toward nuclear
configurations of DVR are correlated in snakes, lizards, crocodiles, and
birds which exhibit terrestrial and/or arboreal habits with active predation
or active avoidance of predation (Butler, 1980). It would appear
that expansion of the forebrain in the ancestral reptiles reflected a growing
sophistication in sensory processes, motor control, and behaviors, supported
by the development of DVR.
Next Section: Dinosaurs
Among Us?