I. Introduction
This chapter provides a general review of the specializations and evolution of
the avian visual system, focusing on the selective pressures which drove the
development of its current form. Since the vast majority of data is
available from studies of the pigeon, our discussion will focus on this body of
literature. Other birds (e.g., owls, falcons, etc.) will be included as
applicable to the particular topic. Scientists interested in the visual
perception and cognition of birds should find it useful to consider what drove
the evolution of the avian visual system. Consideration and application of
such ideas should, at some level, guide investigations of visual search, concept
formation,
et cetera. It is perhaps conceptually more tractable to think that
our avian subjects must see the world (at least somewhat) as we do. But,
we must understand as best we can, armed with psychophysical, anatomical,
cognitive, and other data, how the organism perceives and operates in its visual Umvelt
(subjective world). Familiarity with the process of visual system
evolution in birds can assist in appreciating the biases and capabilities of
avian subjects. This is especially true in the design of experiments in
the artificial situations of the laboratory and interpretation of data derived
from such investigations.
Birds are highly visually dependent
organisms, possessing visual capabilities comparable (and in some cases,
superior) to those of another visually-dependent vertebrate, the primates.
There are many basic similarities in the visual pathways of birds and
mammals. These commonalities in visual systems are rooted both in distant
ancestral phylogenetic relationships and the operation of convergent
evolution.
Unique specializations of avian visual
systems (as compared to primates) include the existence of double cones,
different photopigment absorption spectra, the presence of oil droplets,
centrifugal efferents to the retina, and an emphasis on the collothalamic
(tectofugal) visual pathway. Evidence from several sources indicates
the avian visual system was driven by alterations of the basic reptilian
plan, primarily in support of flight behaviors. The development of
the collothalamic pathways encouraged the development of the avian telencephalon
as the site for more complex social and feeding behaviors.
Some
Terms and Concepts
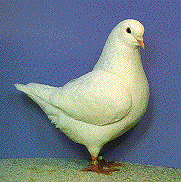
Darwin, in the Origin of Species (1859),
called eyes "organs of extreme perfection." Indeed, many of the opponents
to Darwin's evolutionary theories argued that such a complex entity could
not have arisen from accident, that they must have been divinely "designed",
not driven by a tinkering process occurring over earth's history.
Darwin spent considerable time working out the dynamics of evolution, elucidating
how natural selection could arrive at the "perfection" which naturalists
of the day saw in many biological systems.
The variety of organisms on earth was a
considerable source of both inspiration and data for Darwin's ideas on
how organisms adapted to their environment. The vertebrates which
rely on vision reflect this diversity in the many differences in their
visual systems. The photoreceptors, retinal structure, and other
components of the visual system appear tailored to their unique lifestyles.
However, despite the array of ways in which organisms employ vision in
their survival, there are remarkable similarities in the underlying plan
of how these visual systems operate.
The underlying similarities found in
the diversity of organisms which employ vision are fundamentally driven
by stimulus-organism interactions. From the pineal eyespot under
the skin of amphibians to capabilities of the primate eye, the evolution
of visual functions are driven by the content of the photic stimulus (i.e.,
wave and particle properties of light) and the physical and biochemical
constraints of living organisms. This is true of all sensory systems,
and is the fundamental principle on which perceptual systems evolve and
the constraints under which they operate.
Similarities in organisms' visual systems are
also attributable to the common (albeit in some cases long removed) evolutionary
ancestry shared by all vertebrates. In particular, amniotes (reptiles,
birds, and mammals) share some striking similarities in the organization of
their visual pathways. Ascertaining how much of these similarities in
their visual systems derive from common ancestry is a difficult task. The
stem reptiles from which all amniotes derived (known as the order Captorhinida)
became extinct about 300 million years ago (Carroll, 1988). The study of
modern birds, reptiles, and mammals can indicate which brain areas and visual
behaviors are “holdovers” from ancestral reptilian characteristics.
Conversely, important specializations of the brain for vision can be discovered
by comparing birds and primates, which generally have more sophisticated visual
capabilities than most reptiles. Therefore, traits shared by avian and
primate visual systems, but not reptiles, may indicate specializations in the
brain for their excellent visual capabilities. Looking at both the
similarities (driven by the stimulus and constrained by biology) and varieties
(resulting from genetic mutations and natural selection pressures of the
environment) of visual systems enables an appreciation of how visual systems
originated and have changed across evolutionary time.
Clues to the evolution of the avian
brain and visual system must rely on relatively rare fossil finds and cranial
endocasts. One cannot perform experiments to look at the variables
of natural selection operating across evolutionary time (although interesting
simulations have been performed in the realm of computers and artificial
life). The reconstruction of phylogenetic relationships is difficult,
since fossilized remains are either non-existent or yet to be discovered.
This is even more prominent in the evolution of birds, whose delicate bones
do not usually withstand the passing of geologic time. In addition,
the soft tissues do not fossilize, so any reconstruction of the eye or
brain must be based on cranial structure. However, cranial endocasts can be formed naturally by the deposition and petrification of sediments
in the brain cavity. These remains provide some basis for insights
into brain structures and their relative development in extinct organisms.
Other than fossil analysis, the approach of comparative anatomy offers
clues to the origins and adaptations of the brain and visual system which
led to modern forms.
The conditions which existed in the brains of
amniote ancestors are difficult to ascertain. Keeping in mind the possible
convergences due to evolution under similar environmental pressures, anaysis of
the basic features common to all modern amniotes can be enlightening. For
example, some general features of reptilian brains (the closest relatives of
modern birds) may indicate the condition found in ancestral avian forms.
Crocodilians, which appear to have undergone comparatively few major changes
over the course of evolution, are a valuable species with which to compare
birds. Both crocodiles and birds evolved from the archosaurs, or ruling
reptiles. Their phylogenetic relationship is determined
from both fossil evidence and the reconstruction of their lineages through
methods like cladistics. Sets of shared, similar traits are analyzed and
compared; those organisms which numerically possess the greatest number of
shared characters are then considered more closely related (Butler & Hodos,
1996). Organisms are grouped based on the number and kinds of shared
traits they possess. It is assumed in cladistics that two groups of
animals which share a new or evolutionarily “derived” trait are more closely
related to each other than either is to its common ancestor. The common
ancestor would be in possession of an older structure from which the derived
trait evolved. The identification of shared traits are represented in a
tree-like diagram or cladogram. Each branch reflects the emergence from an
ancestral form of a group having new, derived characteristics not present in the
earlier group. Caution must be taken, however, not to fall into the scala naturae
fallacy. This view of evolution is one of a continuous, escalating
progression from simpler "primitive" forms to more complex and
advanced ones (Butler & Hodos, 1996). Phylogenetic scales, a kind of
Aristotelian staircase from fish and amphibians, through reptiles and mammals,
to the “pinnacle” of evolution, modern man, is simplistic, anthropocentric,
and clearly false based on current scientific data. No living reptile can
constitute a “primitive” form from which birds derived, just as no modern monkey
or ape can represent a direct evolutionary stage leading to humans.
Modern organisms are not primitive
forms of others; all extant organisms have evolved to survive under their
unique selective environmental pressures.
In discussing traits of the ancestral
amniote brain, and how it has subsequently changed through evolution, a
distinction is made between homology and homoplasy. Characteristics which
appear similar in structure and/or function in two organisms may derive from the
condition of the presumed ancestor of the two forms. This is referred to
as homology, which has been defined by Campbell and Hodos (1970): "(structures
and characters) are homologous when they could, in principle, be traced back
through a genealogical series to a stipulated common ancestral precursor
irrespective of morphological similarity."
Aside from homology,
one must keep in mind that convergence due to similar
environmental demands over time can lead to remarkable similarities between
two organisms. Many basic similarities in vertebrate brains may reasonably
be attributed to homology. However, evolution may produce similar
morphologies and other traits in organisms which do not share a close common
ancestor from which the particular trait derived. In these cases,
the trait has risen independently in the two organisms, probably by virtue
of their similar ecological niches and lifestyles. Similar traits
in brain structure or behaviors driven by the adaptation to selection pressures,
but not derived from common ancestry, are referred to as homoplastic.
For example, homoplasy exists when comparing the relationship of a human
hand to that of a raccoon. The raccoon hand resembles a human hand,
but their common mammalian ancestor most likely possessed a forepaw, like
that seen in rodents (Butler & Hodos, 1996). Thus, the similarities
seen in raccoon and human hands probably came about due to convergence,
with both species independently undergoing changes in forepaw structure
in order to better use them as means to manipulate their enviornment.
Traits which two organisms share can also be analogous. Such
characters may have quite different morphology and phylogeny, but serve
very similar functions (Butler & Hodos,1996). For example the
wings of both a dragonfly and a bat are analogous since they are used for
flight, but is very unlikely they share any common morphological ancestry.
Hence, the determination of phylogenetic relationships is difficult, and
evidence from a wide variety of studies must be brought to bear to arrive
at the best approximation of such relations between organisms.
In the next sections of this chapter, we will
look at the evidence indicating the condition of the ancestral amniote
brain, its evolution in birds and mammals, and consider the similarities
and differences found in the brains and visual systems of modern birds
and mammals.
Next Section: Evolution
of the Amniote Brain